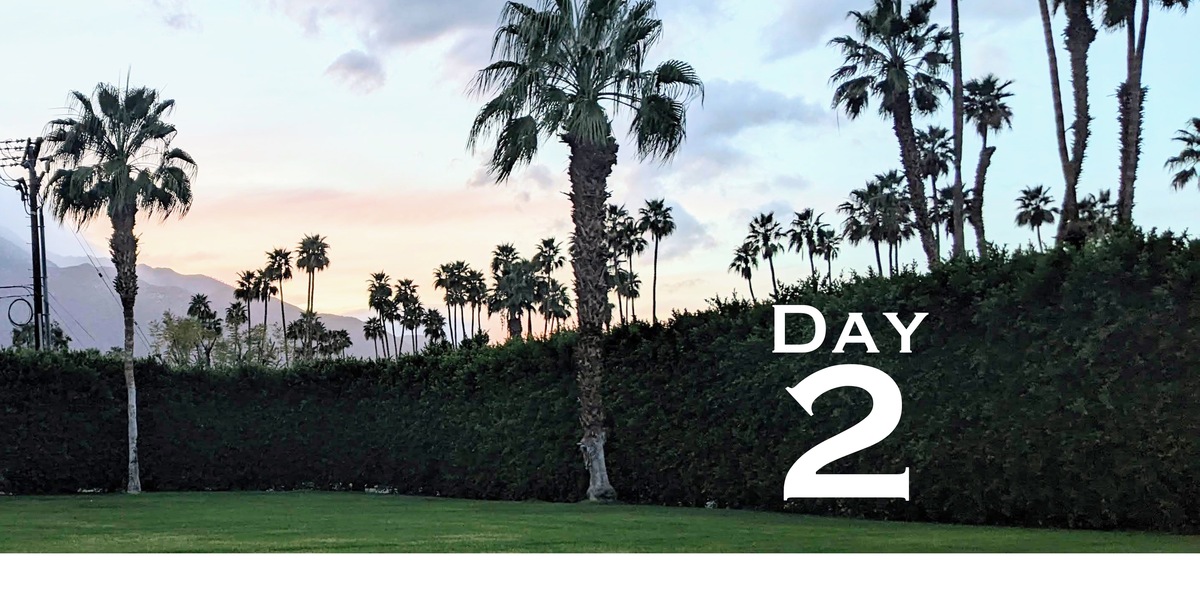
HDBuzz is back for Day 2 of the CHDI HD Therapeutics Conference: Wednesday February 28th in Palm Springs, California. This article summarizes our real-time updates of the conference in community-friendly language.
It’s a brain disease
This morning’s session is titled “It’s a brain disease” and will feature talks about BRAINSSSS! HD scientists are a bit like zombies – they love brains! The session chairs posed big questions to the audience, such as: why does HD affect the brain? Why does it affect certain cells in the brain? And why does HD affect people at the time in their life that it does. This season’s talks tried to address these questions from a variety of different angles.
Christopher Walsh: DNA “signatures” of brain cells in aging and disease
The first speaker of the day was Dr. Christopher Walsh from Boston Children’s Hospital & Harvard Medical School who spoke to us about somatic expansion in the brain. We mentioned that somatic expansion was a hot topic in HD as of late, and many of the talks at CHDI proved that true! Somatic expansion is the increase in the CAG number in some types of cells over the course of someone’s lifetime. There seems to be a link between the amount of somatic expansion and disease progression. Lots of scientists are pursuing this to understand how and why this occurs and also how we might treat it.
Chris’s team look at human brains, generously donated by folks to science. They examine individual cells in those brains, then try to identify single letter changes in the DNA code that are linked to somatic instability.
Over their lifetime, cells accumulate changes in their DNA code, forming “signatures” that scientists can use to classify those cells. Different diseases and cell types have different DNA “signatures,” which researchers can track and categorise. In nerve cells, these changes in DNA signatures happen with age. Chris says this is a bit like a molecular “clock” which gives insight to that person’s degree of aging.
It turns out that the majority of these DNA signature changes are happening in genes that are most important for nerve cells. It also looks like these changes are fairly unique to neurons over other types of brain cells. What’s rather cool about these signatures is that it gives scientists clues about the birth of brain cells, and the common stem cells that gave rise to nerve cells with different DNA signatures. This helps us understand how the brain is formed and how it changes over someone’s lifetime.
Folks in Chris’s team are also using this tracking system to look at how the brain changes in diseases like Alzheimer’s and other neurological disorders, and how this trajectory differs from healthy brains. For example, they were able to use their molecular clock to figure out that brain cells from some people with Alzheimer’s had DNA changes equivalent to around 10 additional years of age compared to people without Alzheimer’s.
Although most of this talk was focused on findings in other diseases, the methods this lab has developed could be an asset to the HD community as scientists continue to pursue DNA repair for HD therapeutics and understand how HD changes the brain.
Mark Bevan: electrical activity in the HD brain
Next on the roster was Mark Bevan from Northwestern University. Mark’s team study HD mice to understand how their brains are affected by the HD mutation, especially an area called the STN, which is thought to suppress movements. Mark recapped some of what we know about CAG repeat expansion: that it may play a role in brain cells getting sick, and that long repeats trigger even more expansion. HD mice with really long CAG repeats have measurable signs of HD – they move and behave differently.
Mark’s lab measures the electrical activity between neurons to understand the activity of different types of cells as mice move around. These techniques involve implanting an electrode into the brain. With this technique, they can get into the nitty gritty of which cells are firing in HD mice and in non-HD mice to unpick exactly what is working and what is going wrong. This is helping them to understand why the HD mice have movement symptoms.
In fact, using special genetic trickery, they were able to make mice without HD move like mice with HD, by stimulating similar patterns of brain cell firing. The challenge is to do the reverse: getting HD mice to move like mice that don’t model HD.
Another interesting finding is that huntingtin-lowering techniques help to restore normal brain activity in some cells but not in others. It also helps to ease the movement symptoms Mark’s team see in the HD mice.
Mark’s work focuses on brain regions that are not normally thought of as top targets for drugs, because they are affected later, or less, in HD. He reminds us that therapies will need to reach widespread areas of the brain. As an electrophysiologist (a scientist who studies electrical brain activity), Mark asks the audience to consider that changes in electrical activity could play a role in damage to neurons. It’s a reminder that a wide variety of techniques give us a clearer picture of changes to the HD brain.
Osama Al-Dalahmah: how HD affects astrocytes
Osama Al-Dalahmah is a neuropathologist based at Columbia University Irving Medical Center. He shared his work looking at how different cells and regions of the brain are affected in HD. There are over 100 different types of cells in the brain! Osama is interested in a type of cell called an astrocyte – a star-shaped cell that helps maintain health and function of neurons.
Osama and his team looked at brains which had been generously donated by folks with and without HD who had passed. There’s been so much data from human tissue this year! This highlights the collaboration between researchers and the HD community. It also gets us closer to understanding HD in the only species we care about – people!
They looked at which genes had been switched on and off in different parts of each brain. They also delved into what was happening at the level of individual cells. Armed with all of this data they could see which genes may track with increasing CAG number and other factors.
The star-shaped astrocytes have genes switched on which suggest they are responding to stress, which we know is happening as brain cells get sick. In fact, Osama’s team found that the more sick brain cells there are, the more the astrocytes are trying to make things better again. As astrocytes work to keep neurons healthy, understanding more about how HD affects them may help us understand how to improve the health of the whole brain.
Osama is trying to identify molecules that might improve the health of both astrocytes and neurons. Its early stages but it looks like his team might have found a molecule that appears to protect HD neurons in a dish!
Matthew Baffuto: understanding CAG expansion through decorations on DNA
Next we heard from Matthew Baffuto from Rockefeller University who spoke about his work on understanding changes to the CAG number in different types of brain cells. One part of this analysis is looking at an area of biology called epigenetics. Essentially, there are inherited decorations on the genetic code that make it easier or harder for a gene to be made into a message or protein.
Matthew’s research uses a special method of sorting out different types of brain cells so they can look at each type in lots of detail and see what molecular changes are happening with disease. As you may have gathered by now, there were a ton of talks using cutting edge technologies to really get into the nitty gritty of what each cell is doing in HD. This is a great example of how HD research benefits from technology developments in other fields.
One of the things Matthew wanted to tease out was which cell types have increasing CAG repeats due to somatic expansion. He looked at the decorative epigenetic markers on genes known to control this, like MSH3 and FAN1, which may sound familiar. The different decorative marks will let Matthew know if levels of MSH3, FAN1, and other DNA repair genes, are likely to be higher or lower in those cell types with somatic expansion. This is a way to examine the cause of lengthening CAG repeats.
Overall, the cells which get sicker in HD had a different array of decorative marks on DNA than cells which are less affected. Many of these decorative mark differences occur on genes known to be important in HD.
Matthew highlighted that changes in these decorative marks aren’t responsible for all the changes in HD in cells. However, his work shines light on how epigenetics can be used to understand how HD affects some drivers of disease, like somatic expansion. We need many different approaches to explain everything that’s going wrong in the cell!
Bob Handsaker – which cells gain CAGs and why?
The final talk of the morning was from Bob Handsaker who is based at Harvard Medical School and the Broad Institute. He studies the very long CAG repeats caused by somatic expansion in the brain. He began by recapping work from Peggy Shelbourne and Vanessa Wheeler who were some of the first researchers to detail that massive expansions can happen in some cells of the brain – up to 1000 CAGs in some cases!
What many researchers are trying to figure out is whether somatic expansion is the main thing that drives HD progression or whether it is involved in another way. Bob’s team uses a fancy type of DNA sequencing which means they can measure CAG numbers up to 1000 CAGs long. Impressive! Bob and his fellow researchers see that somatic expansion really only happens in cell types which get sick in HD. This is one explanation for the vulnerability of certain cells, but it’s a bit different from what other researchers have seen.
Bob shows us a model for how a CAG repeat tract might change from ~42 repeats to 100 repeats and then gets bigger at a faster rate as the person ages. In cells with less than ~150 CAGs, Bob’s team sees very few changes in the genes that are switched on or off. In their analysis, widespread changes only seem to happen when repeats get very large.
They also looked at the speed at which cells gain CAG repeats – it takes decades to reach rapid expansion. By 80 CAGs, it seems cells start gaining more CAGs rapidly, on the order of years. And once they reach 150 CAGs, the cells gain CAGs within a matter of months. In the rapid phase, at 150+ CAGs, the cells become dysregulated. Genes that should be off are turned on and others that should be on are turned off. Bob thinks this leads to toxicity and eventually death of the neurons that undergo this rapid CAG expansion.
Bob and his team are using their model to try and map the trajectory of HD: how many brain cells are lost when different symptoms start to happen? This is a new way of looking at HD and how the disease progresses, enabled by brand new technologies. He shared data from another area called the cortex (the wrinkly outer part of the brain). In these cells they saw a similar two-phase change in CAG numbers – slow and then much faster. This suggests that the process happens across brain regions. It also looks like somatic expansion could be linked to toxic huntingtin protein clumps. Cells which have really long CAGs are also the ones which show clumps.
After so many fascinating talks on somatic expansion, there are some contrasting ideas emerging in the research space. Some scientists believe that CAG repeat expansion is the number one driver of cell vulnerability in the striatum. Others believe CAG expansion kicks off a process that leads to toxicity, but isn’t necessarily the primary player.
Although Bob, Matthew, and other folks have different ideas about what is happening with somatic instability and HD progression, this is not necessarily bad news for the HD research community. In fact this happens all the time in science! Folks have different findings and they battle it out with genuine camaraderie in meetings. Researchers can weigh the strengths and limitations of each piece of data to figure out who might be right. An enthusiastic Q&A followed this talk!
David Altshuler – learning from the Vertex approach to drug discovery
Wednesday afternoon involved a poster session where more than 100 scientists had the chance to present their work to one another, followed by a keynote presentation from David Altshuler, who is the Chief Scientific Officer at Vertex Pharmaceuticals. He is an experienced researcher and pharmaceutical executive who spoke about his work developing drugs for various diseases.
David shared the timeline of discoveries in HD research, mentioning how far we’ve come from the 1980s when some researchers thought we could learn everything we need to know from mice. Slow progress isn’t unique to HD, it’s just really hard to make good medicines for human diseases! Despite tremendous advances, there is still so much we don’t know about human health and disease.
Making new drugs is also super expensive, and many drugs fail during clinical trials, costing time and money to companies and governments, whilst leaving patients waiting without effective treatments. 75% of new medicines fail, and on average it takes more than 12 years to make a drug. That said, David made a key point that it’s encouraging that we have many different technologies available now for the development of medicines. We see this in the HD field with splicing modulators, ASOs, gene therapies and other types of drugs which are all being tested in the clinic – most of these are fairly recent technologies.
As an example of how drug discovery can be successful, he notes Vertex’s efforts on Cystic Fibrosis. The factors that got them to multiple effective drugs are many of the things we already have in the HD field – care centers, natural history studies, and funding agencies that focus on moving research forward. He cited that after 25 years of Vertex working on Cystic Fibrosis, they now have multiple drugs, several of which they developed in just 3 years and can be used in patients as young as 1 month old.
An important point David makes is that genetic therapies should not be the endpoint. These expensive and inaccessible therapies do not serve global patient populations. Vertex is committed to drug modalities which could treat many more people, such as a pill.
David ended with a strong message of hope by saying, “I know this group will find a cure for Huntington’s disease. I don’t know when, but I know you will.”
That’s all for Day 2! Stay tuned for research updates from the last day of the 2024 CHDI HD Therapeutics Conference.